How to calculate bolt yield strength is crucial in engineering. Understanding this vital aspect ensures the safety and reliability of structures and machines. This guide delves into the intricacies of determining bolt yield strength, exploring the underlying principles and practical applications.
From defining bolt yield strength and its significance in engineering applications to exploring various factors influencing its value, this guide is a complete resource. We’ll cover methods for calculating yield strength, practical applications, and illustrative examples to solidify your understanding.
Introduction to Bolt Yield Strength
Bolt yield strength is a critical material property that dictates a bolt’s ability to withstand stress without permanent deformation. Understanding this property is paramount in engineering design, ensuring structural integrity and preventing catastrophic failures. A precise calculation of bolt yield strength is essential for engineers to select appropriate bolts for various applications, guaranteeing safety and reliability. It’s not just about numbers; it’s about ensuring the components of a machine, a bridge, or even a simple fixture can handle the expected loads.The significance of understanding bolt yield strength extends to several crucial aspects of engineering design.
Accurate calculation allows engineers to select bolts with sufficient strength to endure anticipated stresses, minimizing the risk of failure. This, in turn, enhances the safety and reliability of the entire structure or machine. Moreover, understanding yield strength allows for optimal component design, reducing material waste and improving overall efficiency. A thorough understanding of bolt yield strength is a key element in the arsenal of every mechanical engineer.
Material Properties and Bolt Yield Strength
Material properties play a pivotal role in determining the yield strength of a bolt. Different materials exhibit varying responses to stress, leading to different yield strengths. Factors like alloy composition, heat treatment, and manufacturing processes all contribute to the ultimate yield strength a bolt can achieve. Engineers must meticulously consider these factors to select the appropriate material and ensure the calculated yield strength aligns with the intended application.
For instance, a high-strength steel bolt will have a higher yield strength compared to a mild steel bolt, making it suitable for applications requiring greater load-bearing capacity.
Common Bolt Types and Their Typical Yield Strength Ranges
Selecting the right bolt type is crucial for a design. This choice is directly linked to the expected load and the desired safety margin. Different bolt types have different typical yield strength ranges, and it’s essential for engineers to know these ranges. This knowledge allows for informed decisions regarding the bolt’s suitability for specific applications.
Bolt Type | Typical Yield Strength Range (MPa) |
---|---|
Carbon Steel Bolts (Grade 5) | 350-550 |
Alloy Steel Bolts (Grade 8) | 600-850 |
High-Strength Steel Bolts (Grade 10.9) | 850-1050 |
Stainless Steel Bolts | 200-1200 (depending on alloy) |
The table above provides a general guideline. Specific yield strengths may vary based on the manufacturer, specific alloy composition, and manufacturing process. Engineers should always consult the manufacturer’s specifications for precise values.
Factors Affecting Bolt Yield Strength: How To Calculate Bolt Yield Strength
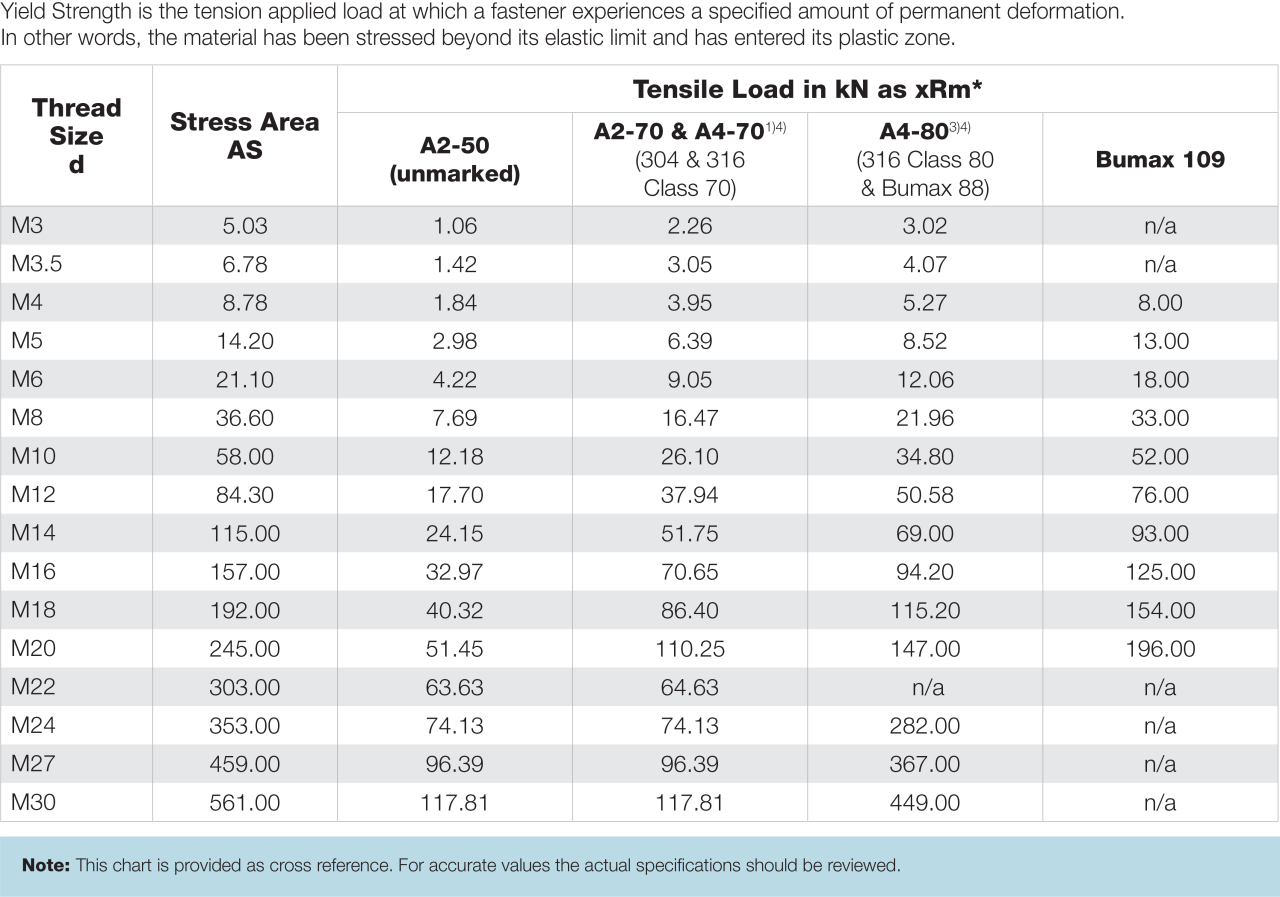
Bolt yield strength, a crucial parameter in structural engineering and mechanical design, isn’t a static value. It’s influenced by a multitude of factors, making a thorough understanding essential for safe and reliable applications. This section delves into the key elements that shape the yield strength of bolts, enabling informed decisions in engineering projects.Understanding these factors allows engineers to select the appropriate bolt material and design parameters to ensure the bolt can withstand the expected loads without yielding.
This meticulous analysis is paramount for structural integrity and safety.
Material Composition
Material composition significantly impacts the yield strength of bolts. Different steel grades exhibit varying strengths. Carbon content, alloying elements, and the presence of impurities all contribute to the ultimate strength and ductility of the bolt material. For instance, higher carbon content generally results in higher yield strength but may also decrease ductility. Alloying elements like chromium, molybdenum, and vanadium can substantially enhance strength and hardenability, improving resistance to deformation.
Understanding the precise composition of the steel is critical in selecting the right bolt for a specific application.
Heat Treatment Processes
Heat treatment processes play a pivotal role in modifying the microstructure of the bolt material, thus influencing its yield strength. Different heat treatments, such as annealing, quenching, and tempering, alter the grain size and distribution of phases within the material. Quenching, followed by tempering, is frequently used to increase the yield strength and hardness of steel bolts. This carefully controlled process leads to a stronger, more resilient bolt capable of withstanding higher stresses.
The specific heat treatment parameters, including temperature and time, directly affect the final mechanical properties of the bolt.
Manufacturing Processes
Manufacturing processes, including forging, casting, and cold heading, also affect bolt yield strength. Forging, a process of shaping metal under high pressure and temperature, can lead to a more uniform microstructure and improved mechanical properties, resulting in higher yield strength compared to casting. Cold heading, a process of shaping metal at room temperature, can also enhance strength but may lead to increased residual stresses.
The selection of the appropriate manufacturing process depends on the desired yield strength, cost considerations, and the specific application of the bolt.
Bolt Dimensions
Bolt dimensions, such as diameter and length, significantly impact the yield strength. A larger diameter bolt typically exhibits a higher yield strength due to the increased cross-sectional area, which can better withstand tensile forces. Similarly, the length of the bolt can affect the stress distribution and potential for localized yielding. Longer bolts may be more susceptible to bending or yielding under high loads, requiring careful consideration of stress concentrations and design parameters.
Yield Strength Comparison of Steel Grades
Steel Grade | Typical Yield Strength (MPa) |
---|---|
ASTM A307 | 250-300 |
ASTM A325 | 827-1034 |
ASTM A490 | 1034-1241 |
AISI 1018 | 290-360 |
AISI 1045 | 480-580 |
This table provides a concise overview of the typical yield strengths associated with different steel grades commonly used in bolts. Note that these values are approximate and can vary based on specific manufacturing processes and testing conditions.
Methods for Calculating Bolt Yield Strength
Unveiling the secrets of bolt yield strength requires a deep dive into the methods used to determine this crucial material property. Accurate calculation is essential for ensuring the structural integrity and longevity of bolted connections in various engineering applications. This section will illuminate the process, from conducting tensile tests to interpreting stress-strain curves, and will highlight the standards that guide these calculations.Understanding bolt yield strength is paramount to engineering design.
A thorough understanding of the methods used to determine this value enables engineers to choose appropriate materials and design robust and reliable structures. It ensures safety margins are met and prevents potential failures.
Tensile Test Method
The tensile test method is a cornerstone in determining the yield strength of bolts. It involves subjecting a carefully prepared bolt specimen to a controlled tensile load, measuring the resulting elongation. This process provides invaluable data for characterizing the material’s response to stress.
Procedure for a Tensile Test on a Bolt Specimen
A standard tensile test procedure is rigorously followed. A precisely machined bolt specimen is secured in a testing machine. The machine applies a gradually increasing tensile load while simultaneously recording the corresponding elongation. The load and elongation data are meticulously logged at regular intervals. This systematic approach ensures reliable and repeatable results.
Calibration of the testing machine is critical for accuracy.
Stress-Strain Curves and Yield Strength Identification
A stress-strain curve is a graphical representation of the relationship between stress and strain. This curve is crucial for identifying the yield strength. The yield strength is the stress level at which the material begins to deform plastically. Visually, this point is often identified by the onset of a noticeable deviation from the linear elastic portion of the curve.
The point of yielding is often marked by a noticeable change in slope on the curve. The stress value corresponding to this point is the yield strength.
Standards and Specifications
Numerous standards and specifications govern the calculation of bolt yield strength. These standards, like ASTM (American Society for Testing and Materials) and ISO (International Organization for Standardization), dictate the procedures for conducting tensile tests, reporting data, and defining specific terms related to material properties. These standards are essential to ensure consistent and reliable results across different laboratories and industries.
ASTM A325 and ISO 898 are common examples.
Calculating Bolt Yield Strength from Material Properties
After a tensile test, the yield strength can be calculated using the formula derived from the stress-strain curve. The calculation involves determining the stress value corresponding to the yield point. The formula is a fundamental relationship between stress, force, and area. The yield strength is then determined from the measured force and the cross-sectional area of the bolt specimen.
For example, if the force at yield is 10,000 Newtons and the cross-sectional area is 10 square millimeters, the yield strength is 1000 MPa.
Table of Testing Standards and Calculation Methods
Testing Standard | Calculation Method |
---|---|
ASTM A325 | Tensile test following ASTM A325 procedures. Yield strength is determined from the stress-strain curve, specifically the offset method or the 0.2% offset method. |
ISO 898 | Tensile test following ISO 898 procedures. Yield strength is determined from the stress-strain curve, using the offset method or the proof stress method. |
Other Specific Standards | Specific standards may have unique calculation methods or specifications for different bolt types and materials. |
Practical Applications and Considerations
Unlocking the secrets of bolt yield strength is crucial for ensuring the safety and reliability of countless engineering marvels. Understanding how to calculate and apply this critical parameter empowers engineers to design robust structures and machinery, mitigating the risk of catastrophic failure. This section dives into practical applications, emphasizing the importance of safety factors, preload, and fatigue considerations in bolt design.Bolt yield strength isn’t just a theoretical concept; it’s a cornerstone of structural integrity.
By understanding its implications and applying appropriate calculations, engineers can confidently design components that withstand expected loads and environmental stresses, ensuring the longevity and performance of the systems they create.
Bolt Yield Strength in Structural Design
Bolt yield strength is fundamental to structural design, particularly in applications involving tension, shear, and combined loading. Engineers must consider the yield strength to determine the maximum load a bolt can withstand before permanent deformation occurs. This crucial knowledge prevents structural failure and ensures the safety of the entire system.
Examples of Calculating Bolt Yield Strength
Numerous engineering applications rely on accurate bolt yield strength calculations. In bridge construction, for example, calculating the yield strength of bolts joining the main girders is vital for ensuring the bridge’s structural integrity under various load conditions. Similarly, in aircraft design, precise yield strength calculations for bolts connecting components are paramount for ensuring the aircraft’s structural integrity and safety during flight.
In the automotive industry, accurate yield strength calculations for engine mounting bolts are critical to prevent engine failure.
Importance of Safety Factors in Bolt Design
Safety factors are indispensable in bolt design. They account for uncertainties in material properties, loading conditions, and manufacturing tolerances. A safety factor, typically greater than 1, is applied to the calculated yield strength to ensure that the bolt can withstand a higher load than predicted by calculations alone. This margin of safety prevents premature failure due to unforeseen circumstances.
For instance, a safety factor of 1.5 applied to a bolt with a yield strength of 100 MPa would mean the bolt is designed to withstand a load of 150 MPa.
Bolt Preload and its Effect on Yield Strength
Bolt preload is the initial tension applied to a bolted joint. This tension significantly affects the bolt’s ultimate strength and load-carrying capacity. By preloading bolts, engineers can improve the joint’s stiffness and durability. A properly preloaded bolt experiences a higher yield strength compared to a bolt without preload. This is because the preload effectively increases the initial stress in the bolt, which must be exceeded before the bolt yields.
Fatigue Failure in Bolts and its Relation to Yield Strength
Fatigue failure, a common mode of failure in bolts, occurs due to repeated stress cycles exceeding the material’s endurance limit. Bolt yield strength plays a crucial role in fatigue analysis, as it determines the stress range that can cause failure. A bolt with a higher yield strength can generally withstand more stress cycles before fatigue failure.
Accounting for Bolt Geometry in Calculations
Bolt geometry, including diameter, length, and thread configuration, significantly influences the bolt’s load-carrying capacity. Engineers must account for these factors when calculating bolt yield strength. Different geometric configurations will affect the stress distribution and the ultimate strength of the bolt. Sophisticated analysis methods, often using finite element analysis (FEA), can accurately model the stress distribution and provide more precise yield strength estimations, especially for complex geometries.
Table Comparing Safety Factors
Engineering Application | Typical Safety Factor |
---|---|
Bridge Construction | 1.5 – 2.0 |
Aircraft Design | 2.0 – 3.0 |
Automotive Components | 1.2 – 1.8 |
Pressure Vessels | 2.5 – 4.0 |
This table provides a general overview of typical safety factors used in various engineering applications. These values are not absolute and can vary based on specific design criteria, material properties, and the level of risk tolerance.
Illustrative Examples

Unleashing the power of calculation, we’ll now delve into practical examples of determining bolt yield strength. These scenarios illustrate how to apply the theoretical knowledge to real-world engineering problems, highlighting the crucial role of accurate yield strength estimations.
Bolt Yield Strength Calculation for a Specific Bolt Type
Calculating the yield strength of a specific bolt type involves a systematic approach, considering material properties and loading conditions. Let’s analyze a high-strength steel bolt (Grade 8.8) with a diameter of 10mm. This bolt is subjected to a tensile load of 50 kN.
Steps for Calculation:
- Material Properties: Obtain the yield strength value for the specified bolt grade (Grade 8.8) from a reputable material data source. For Grade 8.8 bolts, the typical yield strength is around 800 MPa.
- Cross-sectional Area: Determine the cross-sectional area of the bolt using the formula: Area = π
- (Diameter/2)^2. In this case, Area = π
- (10mm/2)^2 ≈ 78.54 mm 2.
- Stress Calculation: Calculate the stress on the bolt using the formula: Stress = Load/Area. Stress = 50 kN / 78.54 mm 2 = 636.6 MPa.
- Comparison and Conclusion: Compare the calculated stress to the yield strength of the material. Since 636.6 MPa is less than 800 MPa, the bolt is well below its yield point and is predicted to perform within the elastic range.
Calculating Yield Strength for a Different Bolt Type
Analyzing the yield strength of different bolt types requires consideration of various factors like material composition, manufacturing processes, and intended application. Let’s examine a stainless steel bolt (AISI 316) with a diameter of 6mm, under a shear load of 20 kN.
Steps for Calculation:
- Material Properties: Consult material specifications to find the yield strength for AISI 316 stainless steel in shear. This value might differ from the tensile yield strength and is typically lower. Assume a shear yield strength of 250 MPa for this example.
- Cross-sectional Area: Calculate the cross-sectional area using the same formula as before. Area ≈ 28.27 mm 2. Note that the area used in shear calculation may be different depending on the bolt geometry.
- Shear Stress Calculation: Calculate the shear stress using the formula: Shear Stress = Shear Load / Area. Shear Stress = 20 kN / 28.27 mm 2 ≈ 707.5 MPa.
- Comparison and Conclusion: The calculated shear stress (707.5 MPa) exceeds the assumed yield strength (250 MPa). This suggests that the bolt will likely yield under the applied shear load. A redesign or reduction in load is necessary to ensure the bolt’s structural integrity.
Real-World Engineering Problem
In a bridge construction project, the safety of the connection between the supporting beams and the deck is critical. The bolts connecting the components must withstand the dynamic loads induced by traffic and environmental factors without yielding. Accurate calculation of bolt yield strength is essential for ensuring the structural integrity and longevity of the bridge.
Bolt Yield Strength Under Various Loading Conditions
Bolt yield strength isn’t a fixed value; it varies with different loading conditions. Consider these:
- Tensile Loading: The most common loading condition. The tensile load directly influences the stress on the bolt.
- Shear Loading: The load applied parallel to the bolt’s cross-section. Shear strength is often different from tensile strength.
- Combined Loading: A combination of tensile and shear forces. The combined effect must be evaluated. Complex calculations often require numerical methods or software tools.
Stress-Strain Relationship
Understanding the stress-strain relationship for different materials is critical in determining bolt yield strength. The relationship dictates how much stress a material can withstand before yielding.
Stress-strain curves provide a graphical representation of this relationship.
Material | Stress-Strain Curve | Yield Strength |
---|---|---|
Steel | (Illustrative curve showing a clear yield point) | (Value representing the yield point) |
Aluminum | (Illustrative curve showing a gradual yielding) | (Value representing the yield point) |
Titanium | (Illustrative curve showing high strength and stiffness) | (Value representing the yield point) |
Bolt Material Selection
Selecting the right bolt material is crucial for ensuring the structural integrity and longevity of your mechanical assemblies. The chosen material must not only meet the required yield strength but also withstand the specific environmental conditions and operational stresses. Choosing the correct material can prevent costly failures and ensure a robust and reliable design.
Criteria for Material Selection, How to calculate bolt yield strength
Bolt materials are selected based on a multitude of factors. Yield strength is paramount, but other critical considerations include corrosion resistance, fatigue life, and cost-effectiveness. The specific application dictates the optimal material choice. A material that excels in high-temperature environments may not be suitable for corrosive environments.
Factors to Consider in Material Selection
- Yield Strength: The primary driver for material selection, as it dictates the maximum load the bolt can sustain without permanent deformation. This must be sufficient to handle the anticipated loads and stresses in the application.
- Corrosion Resistance: Environmental factors like moisture, chemicals, and salt can significantly affect a bolt’s lifespan. Materials with inherent corrosion resistance or those that can be coated to enhance resistance are vital for long-term performance.
- Fatigue Strength: Repeated loading, even below the yield strength, can lead to fatigue failure. Materials with high fatigue strength are crucial for applications subjected to cyclical stresses.
- Ductility: The ability of the material to deform plastically without fracturing. Ductile materials are often preferred for their ability to absorb energy during impacts and prevent sudden catastrophic failures.
- Cost: The economic aspect is always considered. The cost of the material should be balanced against the yield strength requirements and other critical factors.
- Workability: The ease with which the material can be threaded, cut, or formed into the desired shape.
- Temperature Resistance: For high-temperature applications, specific heat-resistant alloys are required. This factor is critical for maintaining the integrity of the bolts under extreme conditions.
Bolt Material Examples and Yield Strengths
A wide array of materials are used for bolts, each with its own set of properties. The following table presents a few examples, highlighting their respective yield strengths:
Bolt Material | Typical Yield Strength (MPa) | Applications |
---|---|---|
Steel (AISI 1018) | 200-300 | General-purpose applications where moderate strength is sufficient. |
Alloy Steel (AISI 4140) | 600-800 | Applications requiring higher strength, like heavy machinery and critical structural components. |
Stainless Steel (304) | 200-300 | Applications in corrosive environments. |
Titanium | 400-900 | High-strength, low-weight applications like aerospace and demanding industrial environments. |
Inconel | 600-1000+ | High-temperature and high-strength applications, including aerospace, chemical processing, and power generation. |
Selecting the appropriate bolt material is a critical decision, directly influencing the performance, safety, and cost-effectiveness of a design.
Specific Bolt Material Applications
- Steel Bolts (AISI 1018): Common in general construction, light machinery, and assembly applications where moderate strength is sufficient. The lower yield strength compared to alloy steels means they are more affordable.
- Alloy Steel Bolts (AISI 4140): Used in applications demanding high strength and resistance to deformation, such as heavy machinery, bridges, and structural components. Their high yield strength provides superior performance in demanding applications.
- Stainless Steel Bolts (304): Preferred in environments with high corrosion, like marine applications, chemical processing plants, and food processing equipment. Their inherent corrosion resistance makes them ideal for these environments.
- Titanium Bolts: Used in aerospace applications and demanding industrial environments. Titanium’s high strength-to-weight ratio makes it suitable for applications requiring high strength and low weight.
- Inconel Bolts: A common choice in high-temperature applications, such as jet engines and chemical processing equipment. Inconel’s exceptional high-temperature strength and resistance to corrosion make it suitable for extreme conditions.
Epilogue
In conclusion, calculating bolt yield strength is a multifaceted process requiring careful consideration of material properties, manufacturing processes, and design specifications. This guide has provided a comprehensive overview, equipping you with the knowledge to confidently tackle various engineering challenges involving bolt strength. Remember, precision in calculations and adherence to standards are key for successful outcomes.
Common Queries
What are the common types of bolts and their typical yield strength ranges?
Different bolt types have varying yield strengths. A table summarizing common types and their typical ranges will be included in the main content.
How does heat treatment affect bolt yield strength?
Heat treatment processes can significantly alter the microstructure of the bolt material, impacting its yield strength. The guide will explain the specific effects in detail.
What is the role of safety factors in bolt design?
Safety factors are crucial in bolt design to account for uncertainties and potential variations in loading conditions, ensuring the bolt can withstand anticipated stresses without failure. The guide will elaborate on this topic.
How do I account for bolt geometry in yield strength calculations?
Bolt geometry, including diameter, length, and thread configuration, directly impacts the stress distribution and, consequently, the yield strength. The guide will illustrate how to incorporate these factors into calculations.